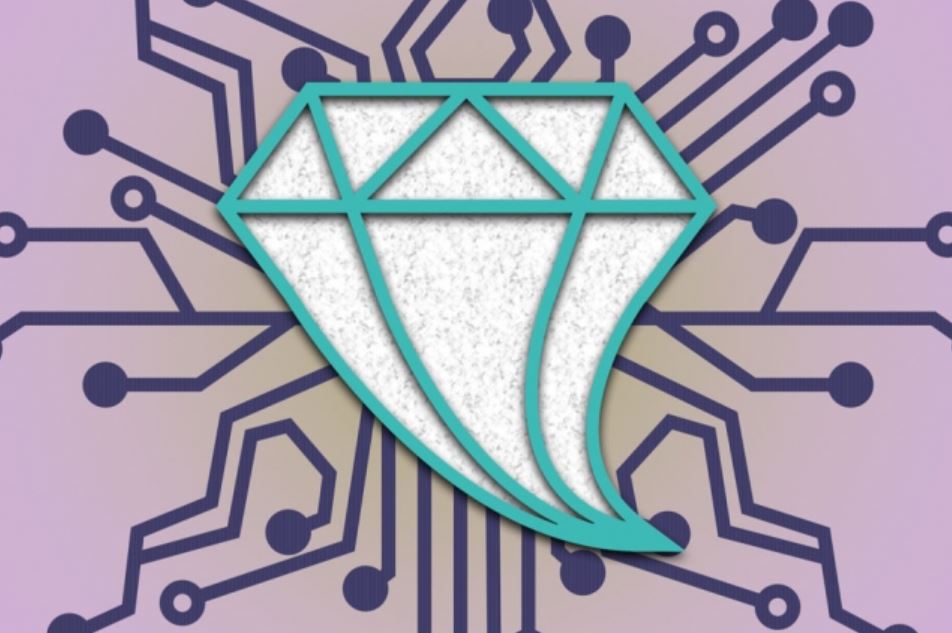
A team of researchers is using AI to facilitate deep elastic strain engineering. In other words, to engineer materials’ properties. Specifically, to change the thermal, electrical, and optical properties of materials.
By applying just a tiny bit of strain to a piece of crystalline material, we can deform the orderly arrangements of atoms in its structure. We can deform the arrangement enough to cause significant changes to its structure, such as the way the material conducts heat. How the material transmits light and conducts electricity may also change.
Scientists from the US, Singapore, and Russia have found ways to use AI to help predict these changes. They say their technology might open up new avenues of research on advanced materials for tomorrow’s high-tech devices.
The researchers wrote about their work in Proceedings of the National Academy of Sciences (citation below). The authors were Ju Li (MIT), Ming Dao (MIT), Zhe Shi (all from MIT); Evgenii Tsymbalov and Alexander Shapeev (both from the Skolkovo Institute of Science and Technology); and Subra Suresh (Nanyang Technological University in Singapore).
Elastic strain can speed up devices
MIT researchers had already incorporated some degree of elastic strain in some silicon processor chips.
In some cases, even a one percent structural change can improve a device’s speed by fifty percent. The change allows electrons to move through the material much faster.
Recent MIT research showed that even diamond can be elastically stretched by up to 9% without failure. This can occur when it is in the form of nanometer-sized needles. One nanometer is one billionth of a meter (10-9 meter).
MIT scientists also showed that nanoscale silicon wires could be stretched purely elastically by over 15%.
Their research, the authors say, has opened up new avenues to determine how to fabricate devices with even more significant changes in the materials’ properties.
Elastic strain made to order
With elastic strain engineering, it is possible to change the properties of a material on the fly. This is not the case with other methods, such as chemical doping, which results in a permanent, static change.
However, the potential of strain-engineered materials has been undermined by the daunting number of possibilities. It is possible to apply elastic strain in any of six different ways. It can happen in three different dimensions – each dimension produces strain sideways or in-and-out. There are also almost infinite gradations of degree. Therefore, it is impractical to explore the full range of possibilities simply by trial and error.
Li, a Professor of Materials Science and Engineering at MIT’s Department of Nuclear Science and Engineering, explained:
“It quickly grows to 100 million calculations if we want to map out the entire elastic strain space.”
Team novel learning methods come to the rescue
This is where the researchers’ novel application of machine learning methods came to the rescue. They provided a systematic way to explore the possibilities. The AI also homed in on the right amount and direction of elastic strain to achieve a specific set of properties for a particular purpose.
Prof. Li said:
“Now we have a very high-accuracy method (that reduces the complexity of the calculations required significantly).”
Prof. Suresh, President of Nanyang Technological University in Singapore, said:
“This work is an illustration of how recent advances in seemingly distant fields such as material physics, artificial intelligence, computing, and machine learning can be brought together to advance scientific knowledge that has strong implications for industry application.”
The authors said their new method could open up possibilities for producing materials tuned precisely for electronic and optoelectronic devices. It would also help in the creation of photonic devices. Specifically, devices for uses in information processing, energy applications, and communications.
Effect of elastic strain on bandgap
The researchers studied the effects of elastic strain on bandgap, a key electronic property that semiconductors have. They studied the effects in both silicon and diamond.
Thanks to their neural network algorithm, they could predict how different amounts of orientations of elastic strain would affect the bandgap. Their predictions were extremely accurate.
According to an MIT press release:
“Tuning of a bandgap can be a key tool for improving the efficiency of a device, such as a silicon solar cell, by getting it to match more precisely the kind of energy source that it is designed to harness.”
“By fine-tuning its bandgap, for example, it may be possible to make a silicon solar cell that is just as effective at capturing sunlight as its counterparts but is only one-thousandth as thick.”
Prof. Li said:
“(In theory, the material) can even change from a semiconductor to a metal, and that would have many applications, if that’s doable in a mass-produced product.”
Sometimes it is possible to induce similar changes by other means. However, these changes generally have several side effects on the behavior of the material. Changing the strain, on the other hand, has considerably fewer such side effects.
Diamond has great potential
As a semiconductor material, diamond has great potential. However, compared to silicon technology, it is still in its infancy. Prof Li said: “It’s an extreme material, with high carrier mobility.” He was referring to how negative and positive carriers of electric current more freely through diamond.
That is why diamond could be ideal for certain kinds of power electronics and high-frequency electronic devices.
According to Prof. Li, by some measures, diamond could potentially perform 100,000 times faster than silicon. However, it has other limitations. For example, nobody so far has worked out a good and scalable way to put layers of diamond on a large substrate.
It is also difficult to ‘dope’ diamond (insert atoms), which is a key part of semiconductor manufacturing.
By mounting the material in a frame that we can adjust to change the amount and orientation of the elastic strain “we can have considerable flexibility” in changing its dopant behavior, said Dr. Dao. Dao is the Principal Investigator & Director of MIT’s Nanomechanics Lab. He is also Principal Research Scientist at MIT’s Department of Materials Science and Engineering (DMSE).
Method is ‘generalizable’ to other aspects, apart from bandgap
This study focused specifically on the effects of elastic strain on the bandgap of materials. However, the method is generalizable to other aspects, the authors wrote. The other aspects not only affect electronic properties but also other properties such a magnetic behavior. They also affect photonic behavior.
We are currently using 1% elastic strain in commercial chips. This team has shown that strains of almost 10% are possible with no fracturing.
Prof. Li said:
“When you get to more than 7 percent strain, you really change a lot in the material. This new method could potentially lead to the design of unprecedented material properties.”
“But much further work will be needed to figure out how to impose the strain and how to scale up the process to do it on 100 million transistors on a chip [and ensure that] none of them can fail.”
Citation
The article will not be online until February 19th, 2019.
“Deep elastic strain engineering of bandgap through machine learning,” Ju Li, Ming Dao, Zhe Shi, Evgenii Tsymbalov, Alexander Shapeev, and Subra Suresh. Proceedings of the National Academy of Sciences (PNAS). DOI: http://www.pnas.org/cgi/doi/10.1073/pnas.1818555116.
Video – Artificial Intelligence
AI stands for Artificial Intelligence. It is the theory and development of software technology that allows machines to perform tasks normally requiring human intelligence. We have ‘natural intelligence’ while robots and other super-smart devices have ‘artificial intelligence.’